Physics
Evaporation
To understand steam we need to first understand what happens to water when it evaporates or boils. When we look at water very closely, we see that it is made of two atoms of hydrogen and one of oxygen. We consider the molecules are bonded together by electrical charges (referred to as the hydrogen bond). The degree of excitation of the molecules determines the physical state (or phase) of water – solid, liquid or gas, ie, ice, water or steam.
Evaporation in an open vesselThe average energy of the particles in a liquid is governed by the temperature. The higher the temperature, the higher the average energy. Within that average, some particles have higher and some have lower energy.
Some of the more energetic particles on the surface of the liquid can be moving fast enough to escape from the attractive forces holding the liquid together. They evaporate. Our particles here are the molecules of H2O and we consider the molecules as bonded together by electrical charges (referred to as the hydrogen bond). The degree of excitation of the molecules determines the physical state (or phase) of the substance. Steam molecules are far more excited than the same molecules in a liquid phase (water) or solid phase (ice).
The diagram shows a small region of a liquid near its surface. Molecules in the water jostle with each other and new molecules gain enough energy to escape from the surface. Notice that evaporation only takes place on the surface of the liquid. If you look at water which is just evaporating in the sun, you don't see any bubbles. Water molecules are simply breaking away from the surface layer.
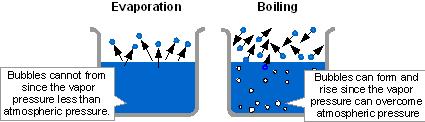
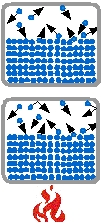
Ordinary evaporation is a surface phenomenon - since the vapor pressure is low and since the pressure inside the liquid is equal to atmospheric pressure plus the liquid pressure, bubbles of water vapor cannot form. But at the boiling point, the saturated vapor pressure is equal to atmospheric pressure, bubbles form, and the vaporization becomes a volume phenomena.
The evaporation of a liquid in a closed containerNow imagine what happens if the liquid is in a closed container. Common sense tells you that water in a sealed bottle doesn't seem to evaporate - or at least, it doesn't disappear over time.
But there is constant evaporation from the surface. Particles continue to break away from the surface of the liquid - but this time they are trapped in the space above the liquid. As the gaseous particles bounce around, some of them will hit the surface of the liquid again, and be trapped there. There will rapidly be an equilibrium set up in which the number of particles leaving the surface is exactly balanced by the number rejoining it. In this equilibrium, there will be a fixed number of the gaseous particles in the space above the liquid. When these particles hit the walls of the container, they exert a pressure. This pressure is called the saturated vapour pressure (also known as saturation vapour pressure) of the liquid.
The effect of temperature on the equilibrium between liquid and vapourIf you increase the temperature, you are increasing the average energy of the particles present. That means that more of them are likely to have enough energy to escape from the surface of the liquid. That will tend to increase the saturated vapour pressure.
Boiling
When water is heated at atmospheric pressure, its temperature rises until it reaches 100°C, the highest temperature at which water can exist as a liquid at this pressure. At this point, the water is saturated with energy. It cannot absorb any more heat while remaining a liquid. Additional heat does not raise the temperature, but converts the water to steam, ie, a 'phase change' occurs. We call this the “
saturation”
point or,
Boiling point and with any further addition of energy, some of the water will boil off as steam.
The boiling point is the temperature at which the vapor pressure of the substance equals the ambient pressure. So, boiling point is dependent on the pressure. At heights, where the atm pressure is much lower, the boiling point is also lower. The boiling point increases with increased ambient pressure up to the critical point, where the gas and liquid properties become identical. The boiling point cannot be increased beyond the critical point. Likewise, the boiling point decreases with decreasing ambient pressure until the triple point. The boiling point cannot be reduced below the triple point.
Intermolecular interactionsIn terms of intermolecular interactions, the boiling point is the point at which the liquid molecules possess enough heat energy to overcome the various intermolecular attractions binding the molecules into the liquid. Therefore the boiling point is also an indicator of the strength of these attractive forces.
Before heat is applied, there is normal evaporation from the surface of the water. In the liquid phase, the molecules are free to move, but are still very close due to mutual attraction, and collisions occur frequently. We start heating the water. More heat increases molecular agitation and collision. As the temperature increases and the water approaches its boiling condition, some molecules attain enough kinetic energy to reach velocities that allow them to momentarily escape from the liquid into the space above the surface, before falling back into the liquid. Further heating causes greater excitation and the number of molecules with enough energy to leave the liquid increases. There is now enough energy to disrupt the attractive forces between the molecules throughout the liquid.
Bubbles of vapour (steam) form within the body of the liquid - those are the bubbles you see when a liquid boils. These steam bubbles float up (the density of steam is much less than that of water) and break through the surface.[Note: The bubbles that precede real boiling in the pot on the stove are either (formerly) dissolved air or water vapor forming on the very hot bottom of the pot that will be condensed before it can get to the top of the liquid.] The space immediately above the water surface is filled with low density steam molecules. There are now more molecules leaving the liquid than entering it. The water is freely evaporating. This is the boiling point.
Once the liquid starts to boil, the temperature remains constant until all of the liquid has been converted to a gas. This 'phase change' requires a tremendous amount of additional energy input. The ability of steam to carry this large amounts of thermal energy is the property that makes it so desirable as a working fluid.
So, how much heat does hot water contain?1kg of water at 30°C has 30 Kcals of heat energy trapped in it; at 100°C it has 100 Kcals of heat energy.
Saturation Curve
Understanding the Vapour pressure or, Steam Saturation curveFor a pure substance there is a definite relationship between saturation pressure and saturation temperature. If the pressure remains constant, adding more heat does not cause the temperature to rise any further but causes the water to form saturated steam. The temperature of the boiling water and saturated steam within the same system is the same, but the heat energy per unit mass is much greater in the steam.
At atmospheric pressure the saturation temperature is 100°C. However, if the pressure is increased, this will allow the addition of more heat and an increase in temperature. The higher the pressure, higher the heat that can be added to the liquid without a change of phase and therefore we reach higher saturation temperatures.
Therefore, increasing the pressure effectively increases both the enthalpy of water, and the saturation temperature. The graphical representation of this relationship between saturation temperature and pressure at saturated conditions is called the vapor pressure curve or, Steam saturation curve (given on page 5).
An egg on a mountain and a pressure cookerThe normal boiling point of water is 100°C. But if you try to cook an egg in boiling water while camping in the Himalayas at an elevation of 10,000 feet, you will find that it takes longer for the egg to cook because water boils at only 90°C at this elevation.
In theory, you shouldn't be able to heat a liquid to temperatures above its normal boiling point. However in a pressure cooker we can decrease the amount of time it takes to cook food. In a typical pressure cooker, water can remain a liquid at temperatures as high as 120°C, and food cooks in as little as one-third the normal time.
To explain why water boils at 90°C in the mountains and 120°C in a pressure cooker, even though the normal boiling point of water is 100°C, we have to understand why a liquid boils. By definition, a liquid boils when the vapor pressure of the gas escaping from the liquid is equal to the pressure exerted on the liquid by its surroundings, as shown in the figure below.
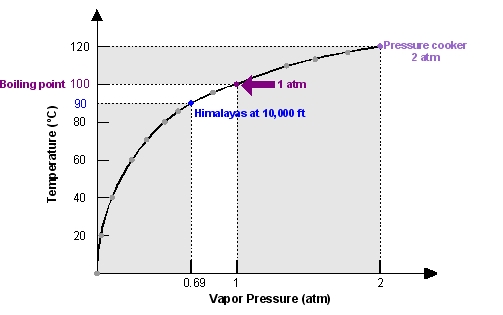
Boiling water in a kettle at MSL The normal boiling point of water at MSL (mean sea level) is 100°C because this is the temperature at which the saturated vapor pressure of water is 1 atm. Under normal conditions, when the pressure of the atmosphere is also approximately 1 atm (or 101325 Pa or 101.325 kPa or 760 mmHg) water boils at 100°C.
Cooking an egg on the Himalayas At 10,000 feet above sea level somewhere on the Himalaya Mountains, the pressure of the atmosphere is only 0.69 atm, 526 mmHg. At these elevations, water boils when its vapor pressure is 0.69 atm, which occurs at a temperature of 90°C. Therefore it takes longer to cook an egg up in the mountains.
On top of Mount Everest the pressure is about 0.256 atm, 260 mbar (26 kPa) so the boiling point of water is 69
°C.
Boiling water in a pressure cooker Pressure cookers are equipped with a valve that lets gas escape when the pressure inside the pot exceeds some fixed value. This valve is often set at 15 psi, which means that the water vapor inside the pot must reach a pressure of 2 atm before it can escape. Because water doesn't reach a vapor pressure of 2 atm until the temperature is 120°C, it boils in this container at 120°C. Therefore food cooks faster in a pressure cooker as it attains a higher temperature by increasing the pressure.
This steam saturation curve is extended for higher pressure towards the right as below:
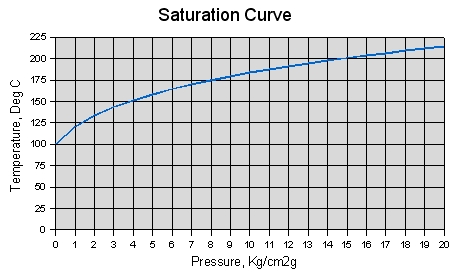
- The blue saturation curve is where the steam/water mix is saturated, or boils at the different conditions of pressure and temperature. Eg, 100°C at 0 kg/cm2g or 170°C at 7 kg/cm2g.
- Above the blue line, the temperatures is above saturation temperature and is called the degree of superheat of the steam.
- Below the blue curve, we have hot water, not steam, and this is called sub-saturated water.
What is the Triple point ? In physics, the triple point of a substance is the temperature and pressure at which three phases (gas, liquid, and solid) of that substance may coexist in thermodynamic equilibrium.
The triple point of water is used to define the kelvin, the SI unit of thermodynamic temperature. The number given for the temperature of the triple point of water is an exact definition rather than a measured quantity.
The single combination of pressure and temperature at which water, ice, and water vapour can coexist in a stable equilibrium occurs at exactly 273.16 kelvins (0.01 °C) and a pressure of 611.73 pascals (ca. 6 millibars, .006037 Atm). At that point, it is possible to change all of the substance to ice, water, or steam by making infinitesimally small changes in pressure and temperature. (Note that the pressure referred to here is the vapor pressure of the substance, not the total pressure of the entire system.)
At this temperature of 0°C or 273K the Enthalpy of water is considered to be 0. Enthalpy of all states are easily found with reference to this triple point.
The bell curve - understanding the phase diagram of steam.
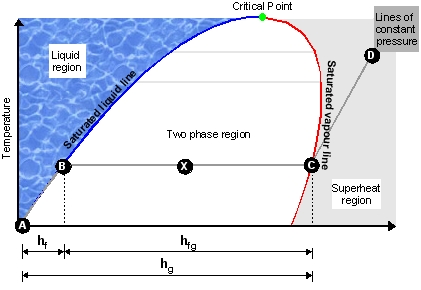
Fig. The bell curve
In this graph, ABCD is a constant pressure line, say 3.5 barg. The other lines on top of it are also constant pressure lines, just at higher pressures. It is called a bell curve because of its characteristic shape.
Assume we have 1kg of water held at a constant pressure. This water will be at atmospheric temperature, say 25°C at point M between points A and B lying on the saturated liquid line.
Enthalpy of water (hf)
We start heating the water. The water shows a change in temperature. Therefore this heat is called Enthalpy of water, liquid enthalpy or sensible heat (hf) of water. Remember, enthalpy is the amount of heat available from any medium in its current state. It moves from point M slowly towards point B. Water starts boiling at point B. Here is where the change of phase from liquid to vapour starts.
Enthalpy of evaporation or latent heat(hfg) Our sample of water, now is a mixture of two phases, water and steam (also called sub-saturated water) and moves along line BC changing into vapour as more heat is applied.
While moving from B to C say at point X, the temperature of the two-phase mixture does not change, as the extra heat applied to this mixture, is being absorbed by the water which evaporates into steam. What this means is that the heat being applied is only being used for a phase change to occur. This heat is the Enthalpy of evaporation or latent heat (hfg).
Like the phase change from ice to water, the process of evaporation is also reversible. The same amount of heat (hfg) that water absorbs to change to steam is released back to its surroundings during condensation, when steam meets any surface at a lower temperature. Steam then changes state from gas to liquid (water) by giving up its Enthalpy of evaporation (hfg) to the process and is called condensate. Thus, Enthalpy of evaporation is the most useful part of heat, as it is the only part of heat which is extracted by the process when steam condenses back to water.
At point C there is no water (liquid) left. We are left with 100% saturated steam or, 100% dry steam. Our water sample has converted to 1 kg of steam. This is the saturated vapour line.
Our aim is to use steam as close to point C, on the saturated vapour line as steam is driest at this point
Superheated steam. If we continue to provide more heat to the steam we move from point C to D. Steam is now 100% dry (dryness fraction =1) and it absorbs the extra heat to show a change in temperature as there is no phase change possible any more. This heat is also 'sensible' heat as the temperature of the steam starts to rise. The steam now is called superheated steam.
Producing Steam at Higher pressure from a boiler. If the steam is able to flow from the boiler at the same rate that it is produced, the addition of further heat simply increases the rate of production. But, if the steam is restrained from leaving the boiler, and the heat input rate is maintained, the energy flowing into the boiler will be greater than the energy flowing out. This excess energy raises the pressure say, to 10 barg, in turn allowing the saturation temperature to rise to 184°C, as the temperature of saturated steam correlates to its pressure. Now we are on the line AB'C'D'.
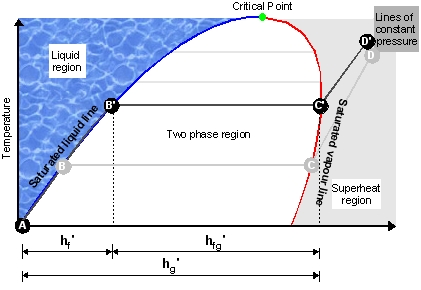
Fig. Bell curve - higher pressure line
So, at pressures higher than atmospheric, more heat must be added to water (hf ' ) before it can turn to steam. Increasing the pressure increases the temperature of the phase change, and increases the total amount of energy ( hg' ) the water/steam can carry. But, the Enthalpy of evaporation ( hfg' ), which is the most useful part of heat, actually reduces!!
Wet steam. In between the saturated liquid line and saturated vapour line - a mixture of steam + water exists. This is wet steam. Only water exists to the left of the saturated liquid line, and only superheated steam exists in the region to the right of the saturated vapour line.
Critical point. The critical point is the highest temperature at which liquid can exist. This is the point at which the saturated liquid and saturated vapour lines meet. The enthalpy of evaporation keeps decreasing as the pressure increases and it becomes zero at the critical point. Water changes directly into saturated steam at the critical point. Above the critical point only gas may exist. In gaseous state the molecules have an almost unrestricted motion, and the volume increases without limit as the pressure is reduced. For steam the critical point is at 374.15°C and 221.2 bara.
Enthalpy of saturated steam, i.e. total heat of saturated steam
The total energy in saturated steam is simply the sum of the enthalpy of water and the enthalpy of evaporation. hg = hf + hfg
Where:
hg = Total enthalpy or total heat of saturated steam (kJ/kg)
hf = Liquid enthalpy (Sensible heat) (kJ/kg)
hfg = Enthalpy of evaporation (Latent heat) (kJ/kg)
In tobacco drying, we start providing heat to dry the moisture laden leaves.
First stage: the process uses sensible heat to raise the temperature of the tobacco. ie, the temperature of process rises.
Second stage: More heat is supplied, but the temperature of tobacco doesn't rise. The water now uses this heat to change state to steam (latent heat) and evaporates from the tobacco.
Steam Tables and how to read them
All of the information just discussed has fortunately been compiled into steam tables. This is the table for the properties of Saturated Steam and Saturated Water. It shows us the correlation of data for various pressures and temperatures. 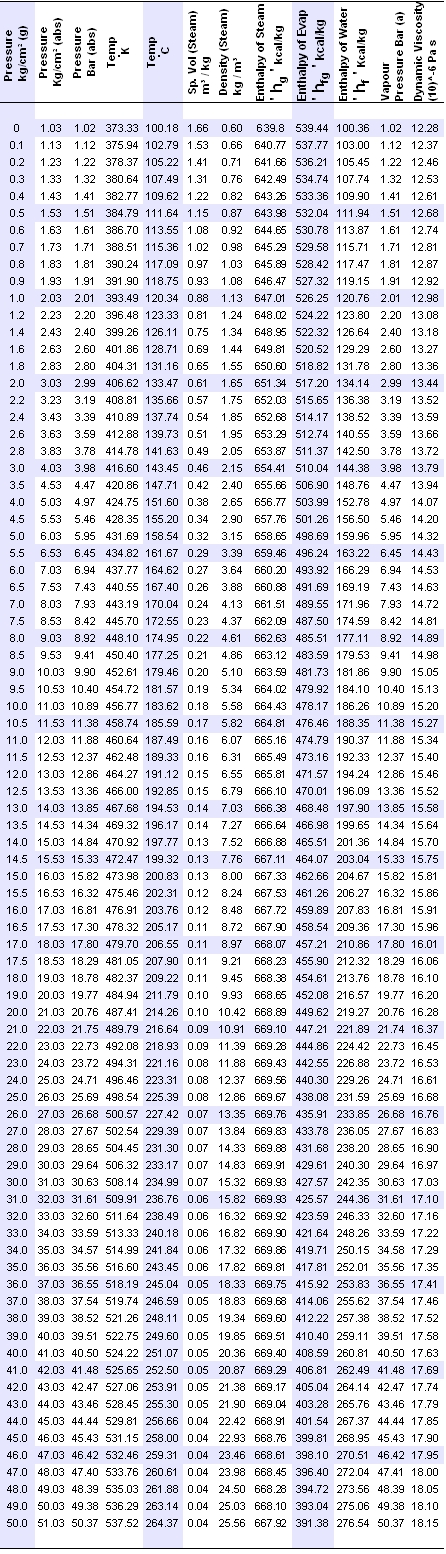
Please make sure you are converting between absolute and gauge pressures as needed.
There are other tables which give you the properties of superheated steam, but we are only concerned with the Saturated steam and water tables, for now.
Examples
Example 1.
1 kg of water heated from 0°C to 100°C contains 100 kcals (100°C - 0°C) per kilogram. To go from 100°C water to 100°C steam at atmospheric pressure requires another 540 kcals per kg. This extra heat which has to be added to convert 1 kg of water to 1 kg of steam is the 'Latent Heat of Evaporation'. This huge energy that water absorbs while changing state is the most important part of steam. This is the the part of heat that steam transfers to process. Remember, while this Latent heat is being added, the water and the steam released are both at the same temperature, 100°C. 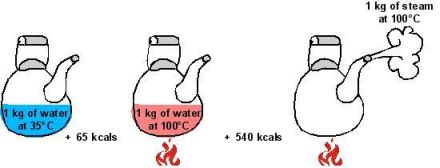
Example 2.
These drawings show how much heat is required to generate one kg of steam at 10 kg/cm2g pressure. Note the extra heat and higher temperature required to make water boil at 10 kg/cm2g pressure than at atmospheric pressure. Note, too, the lesser amount of heat required to change water to steam at the higher temperature. 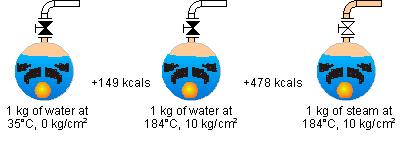
Example 3.
Suppose that we have water vapor (or steam) in a closed container at 120°C and 1 atm. Since the temperature of the system is above the normal boiling point of water, there is no reason for the steam to condense to form a liquid. Nothing happens as we slowly compress the container thereby raising the pressure on the gas until the pressure reaches 2 atm. At this point, the system is at the boiling point of water for that pressure, and some of the gas will condense to form a liquid. As soon as the pressure on the gas exceeds 2 atm, the vapor pressure of water at 120°C is no longer large enough for the liquid to boil. The gas therefore condenses to form a liquid, as shown in the fig above.
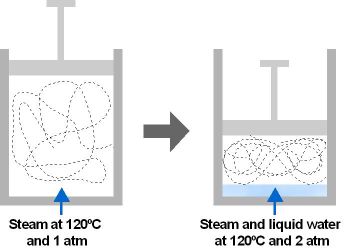
Example 4.
Water boils at 100°C at an atmospheric pressure of 0 kg/cm2 g, and 100 kcals of energy are required to heat 1 kg of water from 0°C to its saturation temperature of 100°C. Therefore the specific enthalpy of water at 0 kg/cm2 g and 100°C is 100 kcals/kg, as shown in the steam tables.
Another 540 kcals/kg of energy are required to evaporate 1 kg of water at 100°C into 1 kg of steam at 100°C. Therefore at 0 kg/cm2 g the specific enthalpy of steam is 640 kcals/kg, as shown in the steam tables.
Enthalpy of steam, hg = 100 + 540 = 640 kcals/kg
However, steam at atmospheric pressure is of a limited practical use. This is because it cannot be conveyed under its own pressure along a steam pipe to the point of use.
FAQs
Question 1. Why is steam generated at higher pressures in a boiler if Enthalpy of evaporation (hfg) drops as pressure increases?
Because of the pressure/volume relationship of steam, (volume is reduced as pressure is increased) generating steam at a pressure of at least 7 barg enables the steam distribution pipes to be kept to a reasonable size.
Question 2. Why does ice float on water?
For most substances, the density decreases as it changes from the solid to the liquid phase. However, H2O is an exception to this rule as its density increases upon melting, which is why ice floats on water.